Sudbury Neutrino Observatory: The Birth of Modern Particle Astrophysics
By Benjamin Tam, June 5, 2019
Article: “Measurement of the rate of nu_e + d –> p + p + e^- interactions produced by 8B solar neutrinos at the Sudbury Neutrino Observatory”
Authors: The SNO Collaboration
Reference: arXiv:0106015 [nucl-ex]
In my regular series, I will be talking about past, present, and future detectors used in particle astrophysics, and the impact these titanic monuments have had on our understanding of the universe. Today, let’s start with the beginning.
In an overview of the state of Dark Matter at the 2017 Topics in Astroparticle and Underground Physics Conference, MIT Professor Tracy Slaytor remarked that the “world is now sailing beyond a new horizon in physics”. After all, we are on the cusp of making fundamental discoveries regarding dark matter and neutrino physics. While many attribute Victor Hess’ discovery of cosmic rays in 1912 as the birth of particle astrophysics, many argue that the modern era of underground mega-detectors came with the surprising – yet problematic – solution to an outstanding issue with the Standard Model.
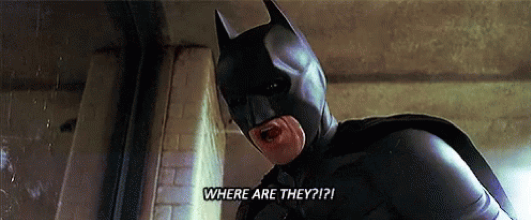
Figure 1: Dr. John Bahcall, the head theorist on the Homestake Experiment, after hearing about the shortage in measured electron neutrinos from the sun. (1970’s, Colourised))
The Solar Neutrino Problem
As early as the 1960’s, early particle astrophysicists operating out of the South Dakota Homestake Gold Mine observed that the number of neutrinos generated by the fusion reactions within the sun fell significantly short of theoretical predictions. The flux of electron neutrinos – the only flavour of neutrinos the sun is capable of producing – was one third the calculated amount. This problem, known as the “Solar Neutrino Problem”, motivated the first generation of underground mega-detectors: GALLEX (Italy), Super-K (Japan), SAGE (Soviet Union), and SNO (Canada).
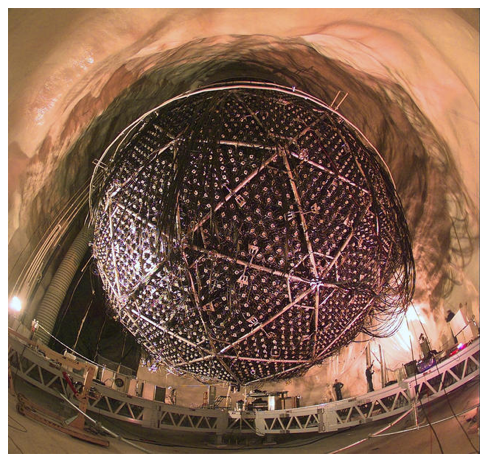
Figure 2: The outside of the massive SNO detector. For additional shielding, the cavity was filled with ultra-pure water soon after this photo was taken
The Sudbury Neutrino Observatory
Of this first quadrumvirate, the Sudbury Neutrino Observatory (SNO) had a unique detection mechanism. Located a water-equivalent depth of 6010m underground, SNO was filled with 1000 metric tons of ultrapure deuterium oxide (D2O), also known as heavy water. As opposed to the liquid gallium in SAGE or the ultrapure water used in Super-K, the use of heavy water allowed for SNO to detect solar neutrinos through the various reactions:
- νe + d -> p + p + e– “Charged Current (CC)”
- νx + d -> p + n + νx “Neutral Current (NC)”
- νx + e– -> νx + e– “Elastic Scattering (ES)”
where d is deuterium, νe are electron neutrinos and νx are neturinos of any of the three flavours (electron, muon, and tau). The additional signals from the NC reactions provided too much of a background for SNO to measure ultra-low energy neutrinos, like those from the primary proton-proton reaction; SNO has to rely on neutrinos from Boron-8 decay, the sixth reaction in the proton-proton chain that accounts for 0.02% of all solar neutrinos. However, the NC and ES reactions provide SNO with a unique advantage over the other mega-detectors in the quadrumvirate: it is sensitive to all flavours of neutrinos.
240.95 live days of data-taking by SNO resulted in the observation of 1169 solar neutrinos from the 3 different reactions, pared down from the 355 million candidate events through dramatic and extensive analysis cuts to guarantee the removal of unwanted backgrounds. From these events, SNO was able to see an ES flux of 2.39±0.34 x 106 cm-2s-1. After accounting for the fact that the ES reaction has a reduced sensitivity to muon and tau neutrinos, the total neutrino flux from all three flavours was determined to be 5.44±0.99 x 106 cm-2s-1. However, the flux seen from CC reactions – sensitive to only electron neutrinos – was merely 1.75±0.07 x 106 cm-2s-1. This implies that nearly exactly 2/3 of the 8B solar neutrino flux were not electron neutrinos! This result was not only the first determination of the total flux of 8B solar neutrinos, but the first ever observation of non-electron flavour solar neutrinos.
Into the future
As the sun’s 8B reaction can only produce electron neutrinos, the fact that all three types were measured coming from the sun was unequivocal evidence for a theory that had been floating around for some time: that neutrinos can oscillate from one type to another. This is only possible if neutrinos have masses – and that the three flavours of neutrinos are made from a combination of 3 “real” neutrinos, each with their own mass.
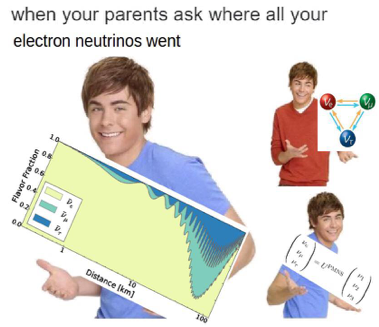
Figure 3: The measurement of 8B solar neutrinos by SNO proved that neutrinos can oscillate from one flavour to another.
Although this solution to the solar neutrino problem won SNO (along with Super-K) the 2015 Nobel Prize in Physics, the fact that neutrinos can oscillate between different flavours opened a Pandora’s box in particle physics. Most critically, the Standard Model predicted neutrinos to have no mass. This additional mass – the first ever instance of the Standard Model being incorrect – opened many new avenues to discovery in a field thought nearly complete. What is the mass scale and hierarchy of neutrinos? How does this affect proposed grand unified theories? Are neutrinos their own antiparticles?
The confirmation of neutrino oscillations by SNO proved the mettle of particle astrophysics and challenged the dominance of the Great Colliders like those at Fermilab and CERN, which previously dominated the field of particle physics. SNO ushered a new era of faith in underground mega-detectors – the bread and butter of particle astrophysics – and paved the way to the explosion of underground experiments in recent years. Many now see these experiments as the best path to addressing outstanding questions in cosmology and particle physics.
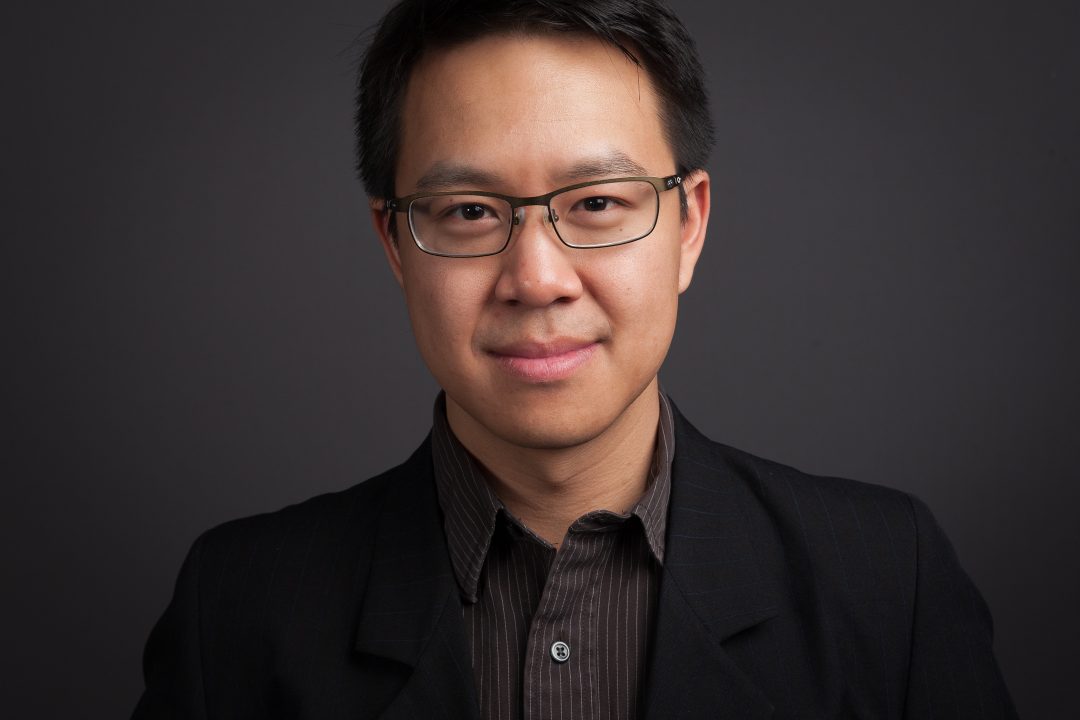
About Benjamin Tam