Would You Like to See A Black Hole?
By Gevy Cao, May 15, 2019
Article: “First M87 Event Horizon Telescope Results. I. The Shadow of the Supermassive Black Hole”
Authors: The Event Horizon Telescope Collaboration
Reference: DOI 10.3847/2041-8213/ab0ec7
On April 10th 2019, the Event Horizon Telescope (EHT) Collaboration made a public announcement on the first ever image of a black hole in the centre of the M87 galaxy. With engineering efforts across the globe, and telescope arrays in a dozen locations all over the world, history was made as the collaboration revealed humanity’s first glimpse of black holes. Would you like to know what it looks like and how they imaged it?
Introduction
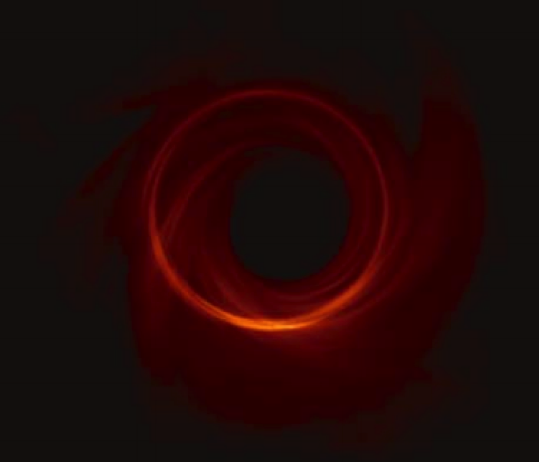
Figure 1a: Figure 4c from the paper showing the simulated event horizon (dark region surrounded by photon emission rings) of the black hole in the centre of the M87 galaxy.
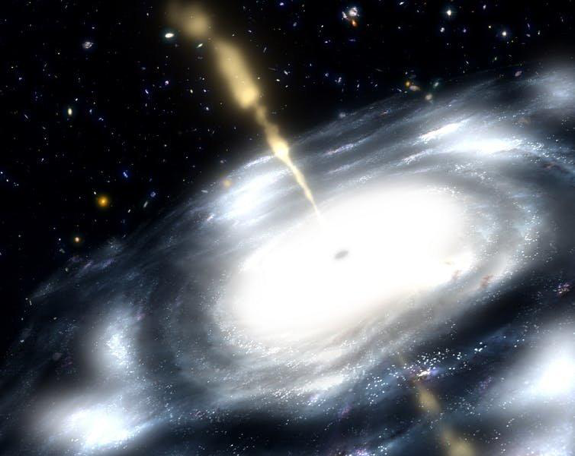
Figure 1b: Taken from K N. Gourgouliatos (link here) show an accretion disk and jet streaming at the heart of a supermassive black hole.
A black hole is a compact mass predicted by General Relativity. One of the main features of a black hole is its event horizon, where nothing, not even light can escape (aka photon capture zone). The event horizon of the black hole can be seen in the simulation image below. The photon emission ring surrounding the horizon originated from the radio waves from an accretion disk and a so-called “jet” (streams of plasma travelling near the speed of light, as can be seen in Figure 1 right). Disks and jets are essential to the imaging of black holes, as you might have inferred – the only way to “see” a black hole is through its surrounding visible features, as the event horizon itself (and the black hole within) is electromagnetically invisible.
Imaging Black Holes 101
An arcsecond
An arcsecond (or as) is a unit commonly used in measuring distant astronomical objects. At the scale of the distance between stars, SI units lose their intuitive meaning. Instead, arcseconds or arcminutes are used to describe the apparent size of an object. An illustration is shown in Figure 2.
The conversion to angles works as such: 1 arcminute = 1’ = (1/60)°; 1 arcsecond = 1’’=(1/60)’. For example, the distance between the M87 black hole and the Earth is about 5.2 x 1020 km, or 55 million light years. The diameter of the photon emission ring of the black hole is ~1011 km, or in the measure of angular diameter, ~40 μas.
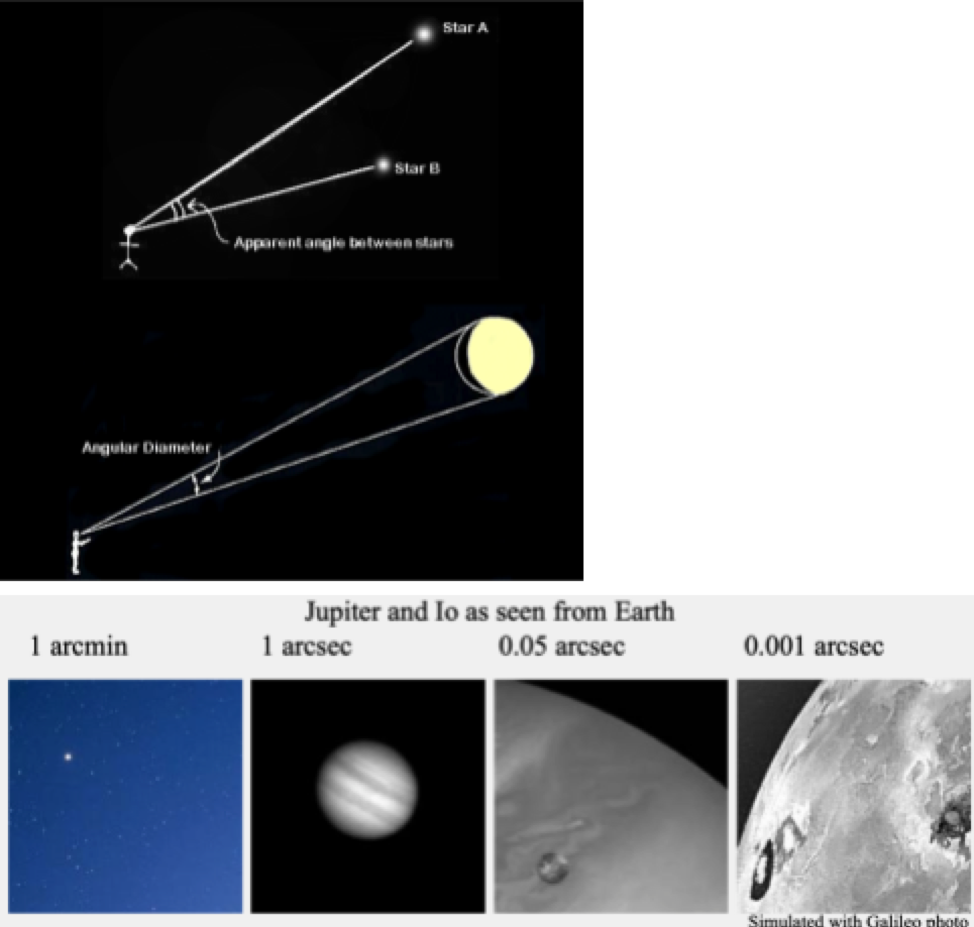
Figure 2: Top: A cartoon illustrating angular diameter and apparent angle between stars (taken from here). Bottom: An example of the angular measurements of Jupiter in arcminutes and arcseconds as seen from the Earth (taken from here).
VLBI (Very Long Baseline Interferometry)
VLBI is an interferometry technique utilizing the size of the Earth to image distant astronomical objects. The angular resolution, θ, of the image of a black hole (or any astronomical object) is given by θ=1.22D/λ, where λ is the observing wavelength and D for a single telescope is the diameter, and for an array of telescopes is the maximum distance between them. In the case of radio waves ( in the order of meters), this separation distance must be at least 1010 m to achieve an angular resolution of 10 μas.
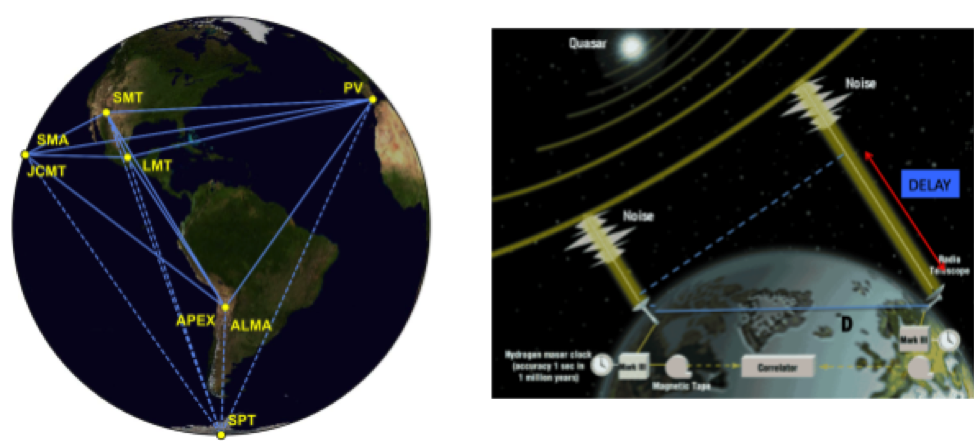
Figure 3: Left: Figure 1 from the paper showing a map of the different telescopes contributing to the EHT observation of M87. Right: An illustration showing the difference in path lengths for light travel to different telescopes in the EHT array. Source
The event horizon telescopes recruited 11 telescope arrays around the world to effectively utilize the diameter of the Earth. Each telescope receives a time delay due to the difference in distance from the source (Figure 3). The telescope stations are equipped with hydrogen maser atomic clocks and the Global Positioning System (GPS) to measure the temporal alignment of the recordings of the black hole. The accuracy in time measurements is within tens of a nanoseconds. The recordings are then correlated in time and space to resolve the signal or “picture” in each frame.
In the future, the constraint from the size of the Earth can be overcome by stationing telescopes some distance away from the Earth.
Image reconstruction
So now you have all the recordings, and assume the correlations are already done for you. How do you actually produce a picture? It’s not a digital camera after all.
The data received at each station needs to be calibrated and processed for the final reconstruction of the image. After aligning the signals in time and space, additional corrections due to residual delays and atmospheric effects in different parts of the world are applied. The final amplitudes and phases of the visibilities V(u,v) — the Fourier components of the brightness distribution of the signals in frequency space (u,v) are fit using image algorithms CLEAN and RML.
CLEAN
When you take a picture of the night sky, you may be able to pick out your favourite star amongst thousands of other stars, if you know what you are looking for. How does an image algorithm know what they are looking for? They do it by “cleaning” (or deconvolving as they call it) the image.
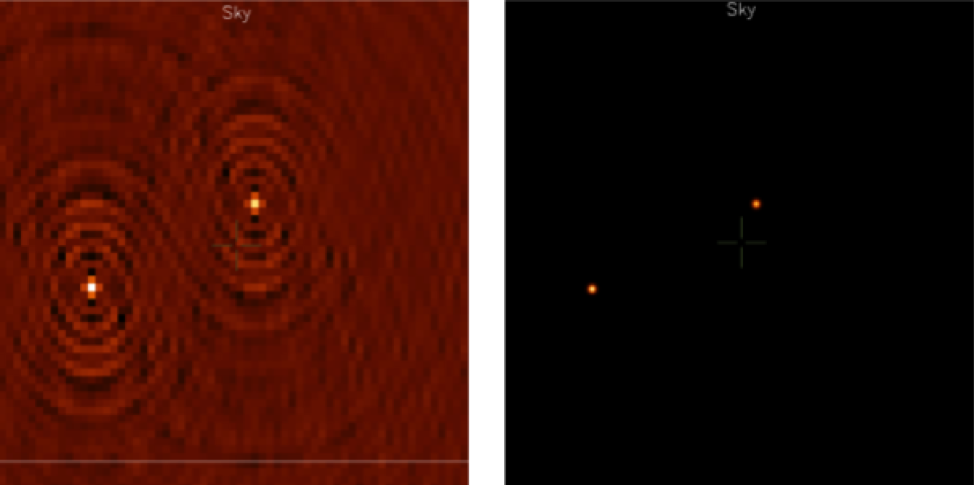
Figure 4: Taken from I. M. Stewart, Deconvolution, Bielefeld, 2012. Left: An example radio array image before processing. RIGHT: the image after processing using CLEAN, assuming point sources (source).
In the UV visibility plane, an image may look like the picture on the left of Figure 4. The algorithm finds the brightest pixel rb in the image I with brightness D(rb), the next image will be processed as I’=I – λ D(rb) B(r-rb), with 0.01<λ<0.2 normally, and B(x) is the beam function. This process is repeated until the brightest value in the image is smaller than some threshold. The deconvolved image is shown on the right of Figure 4:
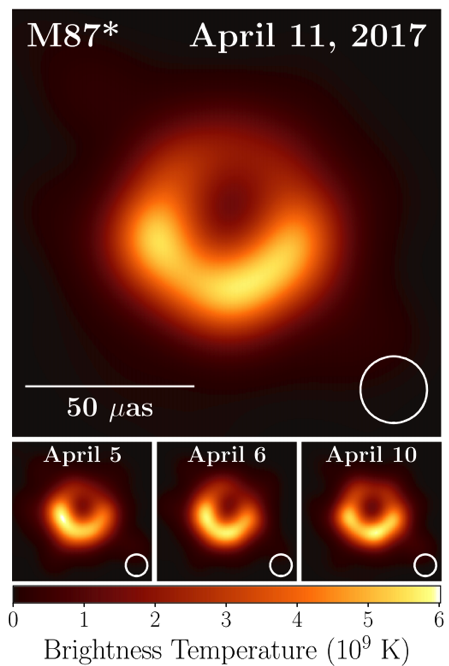
Figure 5: Figure 2 from the paper showing the reconstructed image of the supermassive black hole at the centre of M87.
The RML image algorithm then uses maximum likelihood functions to search for an image that is consistent with observations and favors specific image properties. Here’s the reconstructed infamous image of the M87* black hole:
So anyone with access to telescopes spread across the globe and a computer with image reconstruction algorithms can image a black hole!? I wish! The reality is it is even more difficult than it sounds. Noise in radio receiver electronics, large atmospheric turbulence and decreased telescope efficiencies at shorter wavelengths are all contributing factors to challenges faced by the EHT collaboration. They managed the difficult task, and made possible the direct studies of the event horizon of supermassive black holes via electromagnetic waves, making history by turning the studies of black holes from a mathematical process to a physical one.
In the next couple of years, the EHT collaboration plan on incorporating higher frequencies data and potentially improve the angular resolution by over 30%. A more ambitious long-term goal is to send telescopes to orbit, increasing the separation distance and improving the resolution, as well as more regular observations to capture real-time “movies” of the black hole. The EHT is also expected to be able to study the supermassive black hole at the centre of our own galaxy. Finally, the European Space Agency’s Laser Interferometer Space Antenna program aims to detect gravitational waves emitted by these supermassive black holes in the upcoming decades. The takeaway is: expect a lot of action in the study of black holes, and join one if it interests you – I will be watching the news!
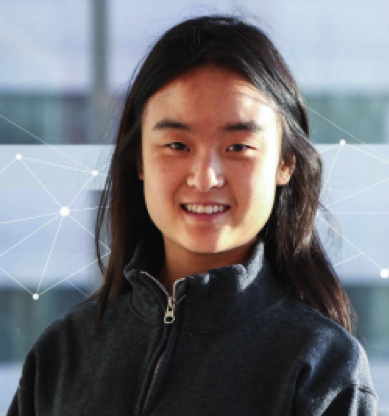
About Gevy Cao:
I am a Master’s student at Queen’s University working on the PICO experiment utilizing bubble chambers for direct dark matter detection. I have worked on a range of tasks from radiation simulations to particle identification using machine learning. You can often find me in contemporary dance studios or martial arts training mats. One of my wildest dreams is to summit Mount Everest.