Searching for Dark Matter, DEAP Underground
By Shawn Westerdale, Apr 24, 2019
Article: “Search for dark matter with a 231-day exposure of liquid argon using DEAP-3600 at SNOLAB”
Authors: The DEAP-3600 Collaboration
Reference: arXiv:1902.04048 [astro-ph.CO]
I guess I’m supposed to start this with “First post!” or something. So there I go, got that out of my system. Now I can talk about this paper…
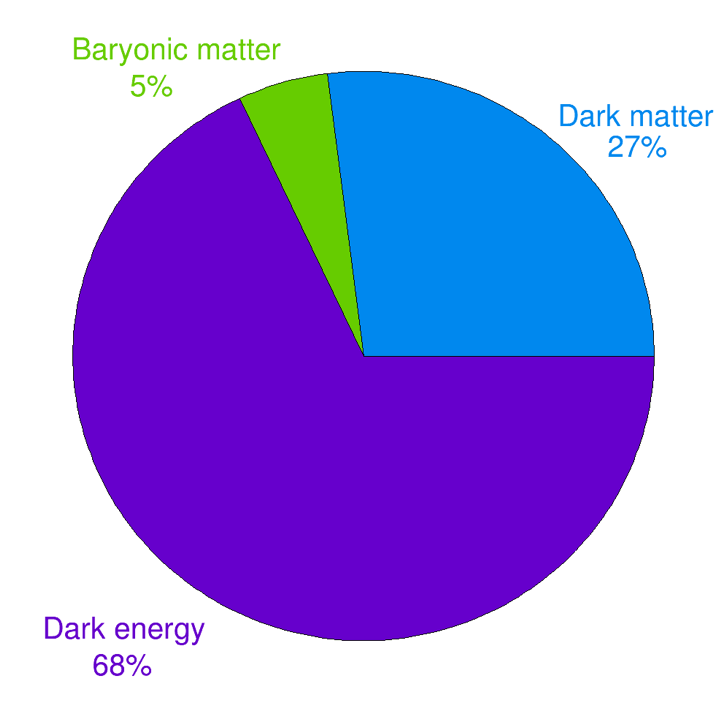
The approximate breakdown of the energy density in the universe, as measured by Planck 2018, showing that there is approximately 5 times as much dark matter than there is baryonic matter.
The approximate breakdown of the energy density in the universe, as measured by Planck 2018, showing that there is approximately 5 times as much dark matter than there is baryonic matter. If you’ve been following from home, you’ve probably heard about the abundance of astrophysical and cosmological evidence that the universe is filled with dark matter. The most recent results from Planck have only strengthened our confidence that dark matter exists in the universe and that there’s a lot of it. (The plot on the right shows the breakdown of the energy density in the universe, as measured by Planck). However, despite all of this evidence for it, we don’t actually know how it fits into the Standard Model of particle physics–that is, we don’t actually know what dark matter is made of, though it is almost certainly some new kind of particle. While there are many extensions to the Standard Model out there that offer dark matter candidates, one particularly popular set of candidates fall under the umbrella of Weakly Interacting Massive Particles (WIMPs). These are dark matter candidates that couple to nucleons with weak-scale (or lower) cross sections and masses on the GeV-TeV scale.
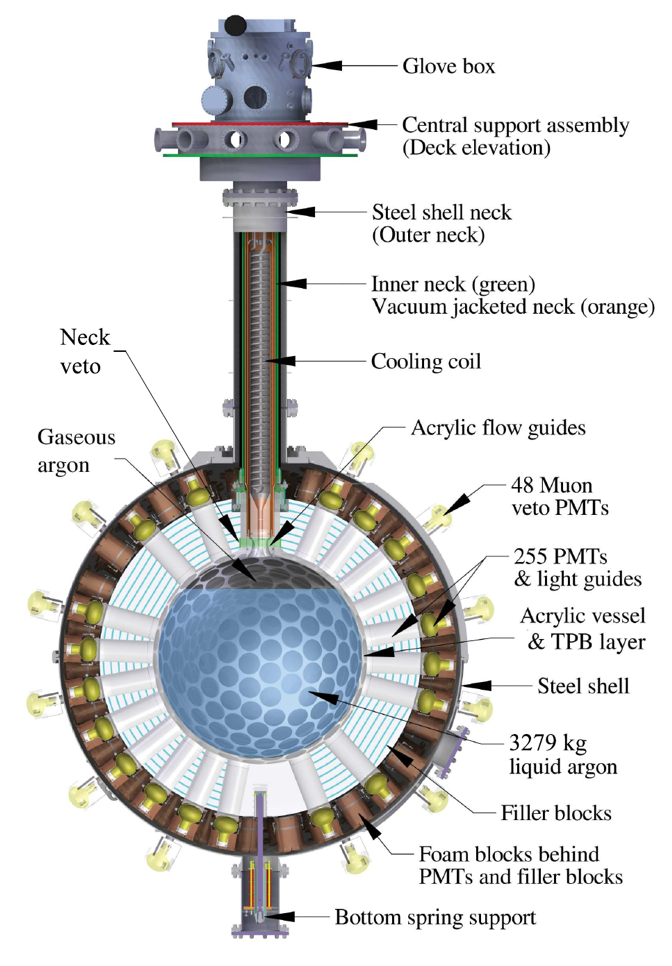
Figure 1 from the paper, showing the cross-section of DEAP-3600.
The DEAP-3600 experiment is one of several experiments currently searching for WIMPs. It’s currently collecting data at SNOLAB, about 2 km underground in an active nickel mine in Sudbury, Ontario. The detector consists of 3.3 tonnes of liquid argon (LAr) contained in an acrylic vessel. The LAr is viewed by a set of 255 photomultiplier tubes (PMTs) that are separated from the LAr by 50 cm of acrylic and foam, mostly in the form of acrylic light guides, which transport light to the PMTs while providing thermal insulation and shielding from backgrounds the PMTs or other detector components. All of this is encased in a stainless steel shell, which is submerged in a large water tank. A set of PMTs mounted on the stainless steel shell, looking outward, detect Cherenkov light produced in the water by passing muons, further suppressing the backgrounds. The detector is illustrated in the imagine on the left, and a more thorough description of the detector can be found in this paper (and even more details here).
The important thing to remember is that dark matter direct detection is like searching for a needle in a haystack. That is–we expect dark matter signals to be extraordinarily rare, which means the vast majority of the signals in the detector will be from backgrounds (things that aren’t WIMPs). To perform a sensitive WIMP search, then, the big challenge typically comes down to modeling and mitigating backgrounds.
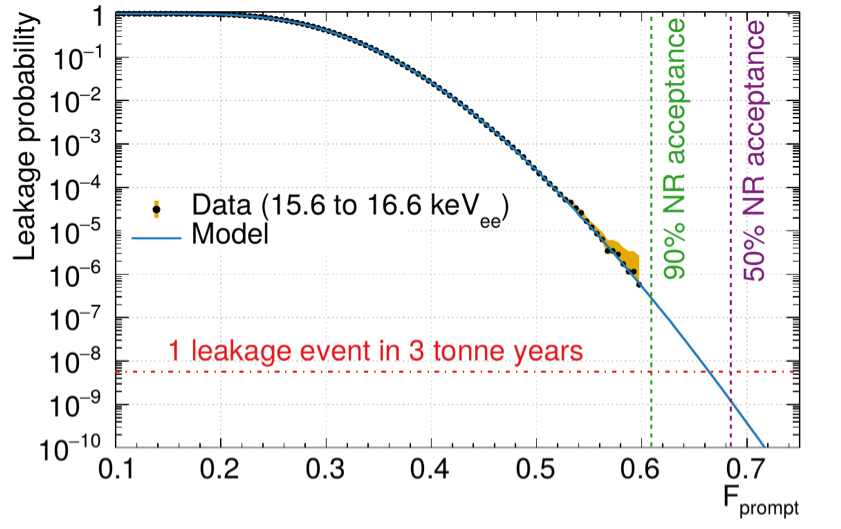
Figure 13 from the paper showing the probability of an electron recoil (ER) being detected above a given Fprompt (the fraction of PEs detected in a prompt window) value in the lowest 1keVee bin in the WIMP-search region of interest. For comparison, vertical lines show the values above which 90% or 50% of nuclear recoils are expected to be found.
The analysis in this paper follow up on the results presented here based on a 4.4 live day exposure taken when the detector first began running. The first half of this paper largely focuses on calibrating the detector to determine the energy scale of the response (they report 6.1 photoelectrons (PE)/keVee (electron equivalent) for a WIMP search window that extends from about 95 PE to 200 PE). By mapping out the hit pattern of photons detected in each PMT, they achieve a position resolution of roughly 3.0-4.5 cm in the WIMP-search energy window. They also demonstrate extremely powerful pulse shape discrimination–the technique of measuring the time profile of a scintillation pulse to determine the nature of the scintillating particle. In this case, most backgrounds come from β and γ-ray interactions, which scatter on Ar electrons. These electron recoils (ERs) scintillate with a very slow time profile. WIMPs, however, are expected to scatter off of Ar nuclei. These nuclear recoils produce much faster scintillation light. As a result, this pulse shape discrimination technique drastically reduces the backgrounds from most backgrounds in a LAr-based detector, leaving only the much rarer backgrounds produced by neutrons and α-particles. The figure to the right shows the discrimination that they achieve in the lowest energy bin used for the WIMP search – the discrimination power becomes greater at even high energies. This is especially important because the LAr in DEAP-3600 is derived from the atmosphere, which means that it has a total activity of about 3.3 kBq of 39Ar in the detector. This isotope β-decays with a 565 keV endpoint, and so strong discrimination is especially important.
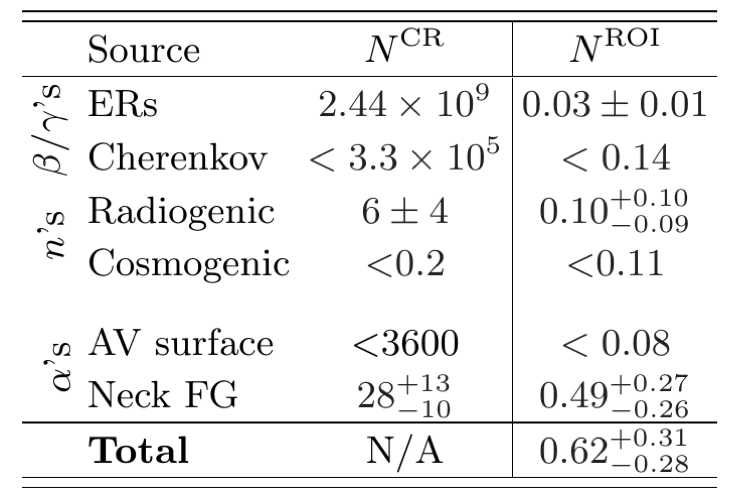
Table 3 from the paper showing the predicted number of events from each background source in its respective control region (CR), NCR and the total number in the WIMP region of interest (ROI) after applying both fiducial and background rejection cuts, NROI. Upper limits are quoted at 90% confidence level
The rest of the background model and the cuts designed to mitigate the backgrounds are described in the paper. The table on the left summarizes these results. As expected, the electromagnetic backgrounds are handily removed by measuring the events’ pulse shapes. The most problematic background source they mention come from α-decays in the neck of the acrylic vessel. This is the opening at the top of the detector from which the detector was filled. If an α-decay occurs from some trace isotope on the surface of the acrylic structures within the neck, it may scintillate in a thin film of LAr. While these events are at high energies, far above the WIMP search region, the topology of the neck shadows these events so only a small fraction of the light is collected and they appear at low energies. A series of cuts was designed to mitigate these backgrounds, but they came at a high cost in WIMP acceptance.
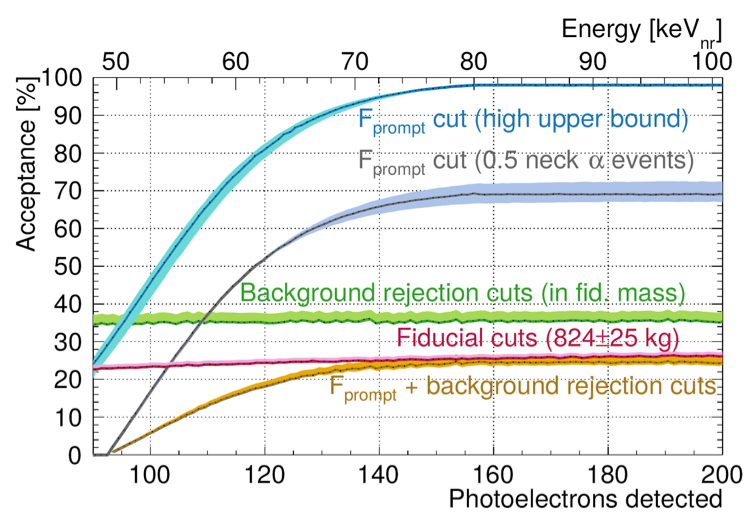
Figure 20 from the paper showing the WIMP acceptance as a function of PE, broken down by cut type.
The acceptance of the WIMP search is summarized in the plot to the right. The Fprompt (the fraction of PEs detected in a prompt window) cut is the cut on the pulse shape that removes electronic recoil events and effectively sets the energy threshold (keeping in mind that due to the relatively low A for Ar, WIMPs tend to produce higher energy signals than they do on heavier nuclei). The fiducial cuts primarily mitigate α-decays on the acrylic vessel surface and neutrons coming from outside the detector (produced by nuclear reactions from trace uranium and thorium decay chains in detector components). The background rejection cuts also help mitigate these backgrounds, but their acceptance is most strongly determined by the cuts used to remove the α-decays in the neck.
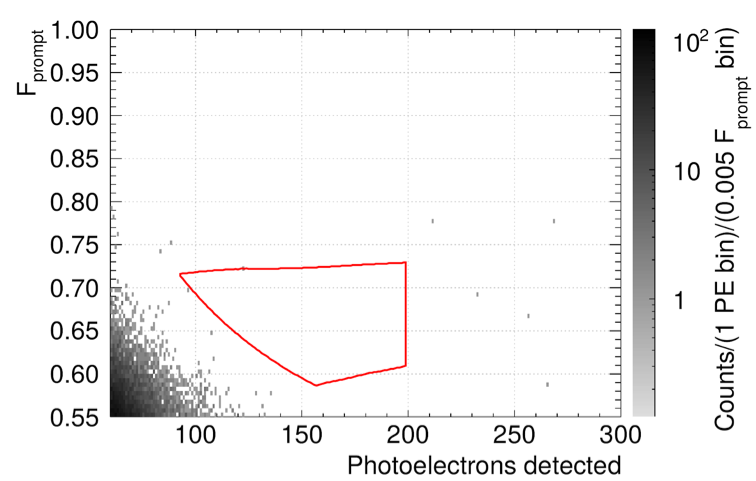
Figure 21 from the paper showing the observed Fprompt vs. PE distribution after all cuts. The region of interest is shown in red.
When all is said and done they report 0 events remaining in the WIMP search region of interest, as shown to the left, showing that dark matter has once again eluded detection. However, based on this non-observation, they were able to place an upper limit on the WIMP-nucleon scattering cross section, under standard assumptions of the nature of the coupling and the velocity and density distribution of the WIMP halo.
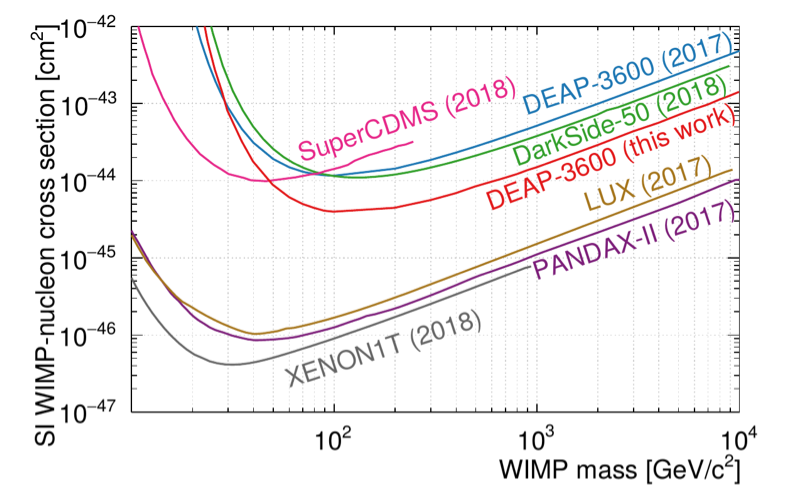
Figure 23 from the paper showing the 90% confidence upper limit on the spin-independent WIMP-neutron cross sections based on the analysis presented in this paper (blue), compared to other published limits, including our previous limit, SuperCDMS, DarkSide-50, LUX, PANDAX-II, and XENON1T.
The excluded cross sections (as a function of WIMP mass) are shown below. This constitutes the most sensitive WIMP search performed to-date using a LAr target for WIMP masses above 30 GeV/c2.
As you probably noticed, the three exclusion curves on this plot that exclude the lowest cross sections are from liquid xenon-based detectors. This is largely due to the larger size of xenon nuclei enhancing the standard spin-independent nuclear scattering cross section. So why search for WIMPs using LAr at all? Well, as a phenomenologist will tell you, the standard assumptions that we make about the nature of the WIMP-nucleon coupling are far from the only possibilities. In order to actually learn anything about the properties that WIMPs do or don’t have, it is necessary to compare observations or non-observations from experiments with different target nuclei. This is exemplified well by this paper by C. Yaguna (arXiv:1902.10256), which shows that for certain subsets of WIMP theories in which the WIMP-nucleon coupling constant differs in sign for protons and neutrons, new regions of parameter space are probed by these results.
The other reason to build detectors using LAr is the low background capability allowed by pulse shape discrimination. LAr detectors trade a larger nucleus for these extremely low background rates, which may especially pay dividends for future larger detectors.
At the end of this paper, DEAP notes that they are continuing to take data, having switched to a blind analysis scheme moving forward for the future. And so as we push ever DEAPer in the hunt for dark matter, we will keep looking until one day, if we are lucky, we might finally find it.
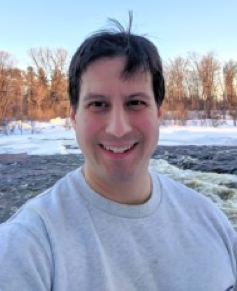
About Shawn Westdale
I am a postdoctoral fellow at Carleton University in Ottawa, working on the DEAP-3600 direct dark matter detection experiment. I completed my PhD at Princeton, working on the DarkSide-50 experiment. And before that, I worked on the MiniCLEAN experiment for my bachelors at MIT. As a hipster physicist, I was into dark matter detectors when they were still underground.